Anacardic acid (6-pentadecylsalicylic acid) induces apoptosis of prostate cancer cells through inhibition of androgen receptor and activation of p53 signaling
Abstract: Anacardic acid (AA) is a mixture of 2-hydroxy-6-alkylbenzoic acid homologs. It is widely regarded as a non-specific histone acetyltransferase inhibitor of p300. The effects and the mechanisms of AA in LNCaP cells (prostate cancer cells) remain unknown. To investigate the effect of AA on LNCaP cells, we had carried out several experiments and found that AA inhibits LNCaP cell proliferation, induces G1/S cell cycle arrest and apoptosis of LNCaP cell. The mechanisms via which AA acts on LNCaP cells may be due to the following aspects. First, AA can regulate p300 transcription and protein level except for its mechanisms regulating function of p300 through post-translational modification in LNCaP cells. Second, AA can activate p53 through increasing the phosphorylation of p53 on Ser15 in LNCaP cells. AA can selectively activate p21 (target genes of p53). Third, AA can down-regulates androgen receptor (AR) through supressing p300. Our study suggests that AA has multiple anti-tumor activities in LNCaP cells and warrants further investigation.
Key words: Prostate cancer; anacardic acid; apoptosis; LNCaP; p300; p53
Introduction
Many plants have been applied for the treatments of cancers (1). Tand have shown certain therapeutic effects, both in vitro or in vivo (2,3). Anacardic acid (AA) is a mixture of 2-hydroxy-6-alkylbenzoic acid homologs and is commonly found in Anacardiaceae family (4,5). It has been widely recosnized as a non-specific histone acetyltransferase inhibitor of p300 (6). Meanwhile, it has shown certain anti-tumor activities (7,8) by inhibiting histone acetyltransferase (9-13) and transcriptional factor nuclear factor-κB (14) in cell and animal models. In addition, it may efficiently inhibit the proliferation of breast carcinoma cells (15).
Prostate cancer (Pca) has a high mortality, ranking second only next to lung cancer (16). Androgen is an important biological effector of prostate cancer progression (17). Many studies have demonstrated that phytochemicals can effectively inhibit the apoptosis and proliferation of prostate cancer cells (18-22). Chun et al. reported inhibition of cell viability and apoptosis of Pca cells treated by andrographolide, which is extracted from a medicinal plant (23). Although the anti-tumor activity of AA has been confirmed in numerous cancers including prostate cancer, the mechanisms remain unknown.
In this study, we explored the role of AA for LNCaP cells. In the investigation of the mechanism, we used siRNA against the p300 to inhibit p300 gene in LNCaP cells, and to determine the effects of p300 on other genes closely related to prostate cancer such as AR and p53.
Materials and methods
Reagents
A 50-mmol/L solution of AA (Merck, Germany) was added in dimethylsulfoxide. AA was prepared in dilution with culture medium when necessary. DMEM, growth factor-reduced matrigel and fetalbovine serum (FBS) were bought from BD Corporation (San Jose, CA). The Lipofectamine™ 2000 and the Total RNA Extraction kit were purchased from Invitrogen (Carlsbad, CA). The MTT Cell Proliferation and Cytotoxicity Assay kit and the Annexin V-FITC & PI Apoptosis Detection kit were purchased from KeyGen Biotech (Nanjing, China). SYBR Green PCR Master Mix were purchased from Fermentas (Burlington, Ontario, Canada). The Total Protein Extraction kit was purchased from ProMab (Changsha, China). Primary antibodies for p300, p21, p53, AR, cyclin D1 and siRNA specifically for p300 were bought from Santa Cruz Biotechnology (Santa Cruz, CA). Primary antibody for phospho-p53 (Ser 15) was purchased from Cell Signaling Technology. RPMI 1640 was purchased from GIBCO.
Cell lines and cell culture
LNCaP was purchased from Yinrun (Changsha, China). LNCaP is a classical metastatic prostate adenocarcinoma cell line, derived from metastatic lymph nodes. LNCaP was cultured with both RPMI 1640 and 10% FBS, and subcultured weekly (37 ℃, 5% CO2).
p300 siRNA transfection
The Lncap cells were seeded into 6-well plates (4×104-5×104 cells/well) and cultured in 2 mL basic culture medium containing 10% FBS overnight until the cells were 70% confluent. Cells were transiently transfected with a validated scrambled control siRNA, or p300 siRNA by using InterferinTM transfection reagent. The mixture of siRNA and InterferinTM transfection reagent was incubated for 10 min, added to each well of the 6-well plates and incubated at 37 ℃ for 24 h before drug treatment.
Detecting the inhibition of cell growth using MTT assays
Lncap cells were treated with AA or transfected with p300 siRNA before drug treatment. Cells were incubated at various concentrations (0, 5, 25, and 125 μmol/L) at a series of time points (0, 4, 24 and 28 h). Then, 5 mg/mL of MTT solution (10 μL, Yinrun, Changsha, China) was carefully added to each plate well. Then, the LNCaP was cultured for another 4 hours. All media were subsequently discarded, and plates were read at 492 nm (A value) with the addition of 150 μL of DMSO in each well. All tests and determinations were repeated in triplicate. The survival rate was calculated by subtracting the background OD value (complete culture medium without cells) from the OD value from each test well.
Detection of cell cycles using flow cytometry
LNCaP was incubated in dishes with a density of 1×105/mL. It was incubated with AA at different concentrations (0, 5, 25 and 125 μmol/L) at 30 ℃ and 5% CO2 for 24 hours. The cells were digested with 2.5 g/L trypsase in a shaking bath. Then LNCaP cells were collected by centrifuge (1,500 rpm, 5 min), and re-suspended in 70% ethanol in PBS for 24 h at 4 ℃ for fixation. Afterward they were harvested and ready for analysis of cell cycle by flow cytometer (Becton-Dickinson, Franklin Lakes, NJ).
Detection of cell apoptosis using flow cytometry
A detection of apoptosis of LNCaP cell treated by AA was analyzed by determining the ratio of cells with nucleus concentration and fragment. Cells were collected at 24 h following AA treatment at different concentrations (0, 5, 25 and 125 μmol/L), and then suspended in the buffer. During the apoptosis assay, the cells were stained with PI and annexin V-FITC (Invitrogen, Carlsbad, CA) and determined by flow cytometer.
Detection of mRNA expression using real-time PCR
Total RNA of Lncap was extracted using Trizol (Invitrogen), according to the manufacturer’s instructions. cDNA synthesis was performed with 2 μg of total RNA using RevertAid™ H Minus First Strand cDNA Synthesis Kit (Fermentas, Burlington, Ontario, Canada). The primers (ProMab) were designed by using Primer Express 3.0 software (Applied Biosystems), and their sequences were as follows: p300(275bp), forward 5-CAGCGACTCCTTCAGCAA-3; reverse 5-GCTACCAGTCCAGGATGTG-3; p53(302bp), forward 5-TTGAGGTGCGTGTTTGTG-3, reverse 5-CTTCAGGTGGCTGGAGTG-3; p21(334bp), forward 5-CCCGTGAGCGATGGAACT-3, reverse 5-CGGCGTTTGGAGTGGTAG-3; AR(131bp), forward 5-CGGAAGCTGAAGAAACTTGG-3, reverse 5-ATGGGCTGACATTCATAGCC-3; cyclin D1(283bp), forward 5-GGATGCTGGAGGTCTGCGAGGAAC-3, reverse 5-GAGAGGAAGCGTGTGAGGCGGTAG-3; GAPDH(452bp), forward 5-ACCACAGTCCATGCCATCAC-3, reverse 5-TCCACCACCCTGTTGCTGTA-3. Quantitative PCR was performed using SYBR Green Master Mix with the use of an ABI PRISM 7500 sequence detection system (Applied Biosystems) Amplification data were normalized to the lowest sample, and quantification of gene expression was performed using the ΔΔCT calculation, where CT is the threshold cycle; the amount of target gene, normalized to the lowest sample, is given as 2-ΔΔ CT(x1).
Detection of protein expression using Western blot analysis
After LNCaP was cultured with AA at different concentrations (0, 5, 25 and 125 μmol/L) for 24 hours, quantification of protein expression (p300, p53, p53-ser15, p21, AR and cyclin D1) was assessed by Western blotting. Cells were harvested and cultured in RIPA buffer (15 min, 4 ℃) before the incubation of lysed products (95 ℃, 5 min). We then detected the protein concentration using DC Protein Assay (Biorad Laboratories, CA). Then, 100 μg protein was loaded on a 12% Acrylamide gel before transferring to membranes, which were blocked by PBS and low-fat milk for 1 hour. The blocked membrane was cultured with primary antibodies (60 min, 20 ℃) and then in the secondary HRP-conjugated antibody (60 min, 20 ℃). Protein expressions were determined by luminol-enhanced chemiluminescence (Thermo Scientific, MA) and exposure to a film (Kodak-Industrie, France). Beta-actin was adopted as intra-reference.
Statistical analysis
The software package SPSS 16.0 was applied for statistical analyses. P<0.05 was considered statistically significant. Mainly statistics methods included t test and one-way analysis of variance. Numerical values are represented as mean ± SD.
Results
AA inhibited LNCaP cell proliferation
To investigate the effects of AA on LNCaP cells, we detect the influence of different doses of AA on LNCaP cells using the MTT assay. The cells were treated with 0, 5, 25, and 125 μmol/L AA for 4, 24, and 48 h. As showed in Figure 1, after having been treated by AA with 24 hours, LNCaP cells were insensitive to the 5 μmol/L AA treatment. However, significant inhibition of cell proliferation by 125 μmol/L AA treatment was detected compared with 25 μmol/L (P<0.05), which suggested it highly sensitive to 125 μmol/L AA treatment. Treatment with 25 and 125 μmol/L AA showed similar effects at 24 and 48 h (P>0.05), we chose 24 h as optimal action time. Data was derived from three independent tests.
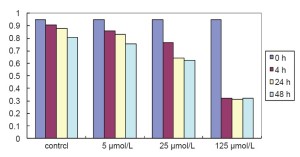
AA induced G1/S cell cycle arrest of LNCaP cells
The impact of AA on cell cycle distribution was measured via flow cytometry. Treatment with 0, 5, 25, and 125 μmol/L AA significantly increased the cell number at G0/G1 phase by 47%, 56%, 59%, and 60%, respectively, and decreased the cell population at S phase by 39%, 38%, 27%, and 29%, respectively (Figure 2). Cells at G0/G1 stages sharply increased after treating LNCaP cells with 125 μmol/L AA for 24 hours.
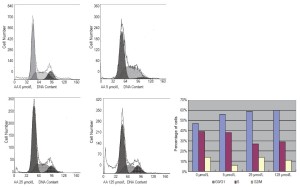
AA induced the apoptosis of LNCaP cell
To investigate whether apoptosis is involved in the influence of AA on LNCaP, we determined the proportion of apoptotic cells using a flow cytometer by double staining of cultures with PI and annexin V-FITC. A sizable increase of apoptosis induced by AA in LNCaP was clearly showed in Figure 3. The proportion of late apoptotic cells at 24 hours following AA incubation increased significantly compared with the control group (P<0.01).
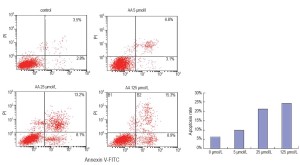
AA down-regulated AR through supressing p300
Analysis of the mRNA and proteins in LNCaP cells treated by AA at various concentrations showed a significant decrease of the expression of p300 and AR in a dose-dependent way. In contrast to AA-treated cells, AR expression was observed in untreated LNCaP cells, and AA reduced the expression of AR and PSA of the cells in a dose-dependent manner (Figure 4). To investigate whether the down-regulation of AR expression was associated with p300, Lncap cells were transfected with p300 siRNA before drug treatment. Real-time PCR and Western blot were performed to determine siRNA effectiveness. We have found silencing p300 using its specific siRNA reduced the mRNA and protein expression of AR as well as p300, indicating that down-regulation of AR by AA is associated with the down-regulation of p300 (Figure 5).
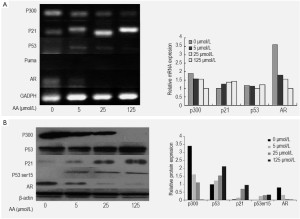
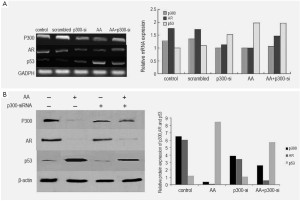
AA up-regulated p53 through phosphorylation of p53 on Ser15
Analysis of the mRNA and proteins in LNCaP cells treated by AA at various concentrations showed significant enhancement of protein expression of p53, but the mRNA expression of p53 was not obvious (Figure 5). From the result of silencing effect of p300 siRNA, we found the effect of p300/p53 signaling to the activation of p53 was little (Figure 5). Meanwhile, treatment with AA resulted in an increased protein expression of phospho-p53 at Ser15 in a dose-dependent manner in Lncap cells. The activation of p53 by AA also enhanced the mRNA and protein expression of p21, a p53-dependent signal mediators, in a dose-dependent manner.
Discussion
The mainstream therapies for prostate cancer remain surgical and endocrine approaches. AA, a natural plant extracts, is considered to be a promising anti-tumor drug with acceptable toxicity and high anti-tumor spectrum. AA inhibits the proliferation of tumor cells in pituitary adenoma (7), breast cancer (15), pulmonary adenocarcinoma, and oral squamous cell carcinoma (24). In our study, the antiproliferation effect of AA on Lncap cells was obvious. LNCaP cells were treated with AA at 5, 2, and 125 μmol/L for 4, 24, 48 h respectively, the inhibition of cell growth and the apoptosis were all significant. AA arrested LNCaP cells at their G0/G1 phase. S phase LNCaP cells decreased, suggesting that AA may have a role in regulating the G1/S phase check point. Moreover, AA exerted such effect in a dose- and time-dependent manner. The optimal concentration of AA was 125 μmol/L, and the optimal reaction time was from 24 to 48 hours. We attempt to explain the role of AA on Lncap cells from the following two aspects.
It is found that AA induced apoptosis and inhibition of NF-κB. In addition, AA can remarkably suppress the activity of IkBa kinase (24). With varying chain length, AA induces cytotoxicity towards several human cancer cell lines in vitro. AA works as an inhibitor of p300, a general transcriptional coactivator and important acetyltransferase (25). p300 remodels chromatin via histone acetylation, resulting in the exposure of the gene promoter and activation of transcription (26,27). p300 can be abnormally activated as oncogene protein inducing tumor cell differentiation. In our study, AA inhibits p300 through supressing p300 transcription and post-translational modification. The inhibition of activation of p300 and acetylation of histone by AA can make the chromosome more condensed and inhibit the combination of oncogenes and its regulator protein (28).
In our study, AA can also down-regulate AR through supressing p300. Androgen is involved in cell cycle progression at G1/S transition and androgen depletion of LNCaP cells resulted in G1 arrest of the cell cycle with suppression of cyclin D1 expression and CDK4 activity (17). Androgen plays a key role in the development of prostate cancer through AR signaling. Aberrant expression of AR, frequently observed in human prostate cancer, has been linked to prostate cancer progression. p300 is key components of the AR transcriptional complex. AA, an inhibitor of p300, can supress AR and its target genes, which can induce cells cycle arrest and apoptosis of Lncap cells through AR signaling. In our study, the suppression of AR expression induced by AA was blocked by p300 siRNA transfection in LNCaP cells, which may indicate that p300 play a key role in the down-regulation of AR expression induced by AA in LNCaP cells.
In addition, the CH1 region of p300 has ubiquitin ligase activity with p53. p300 has a role in degradation of p53 proteins involving ubiquitin through a mechanism of p300/Mdm2-dependent or not (29). p300 can also directly acetylate p53. However, in our study, AA, as an inhibitor of p300, the role of the activation of p53 through p300/p53 signaling was little. Blocking p300 with p300 siRNA transfection did not block the up-regulation of p53 expression induced by AA. Which may because the activation of p53 was mainly caused by AA-induced phosphorylation of p53 on Ser15 rather than its acetylation. AA directly activates p53 and facilitates the phosphorylation of p53 at Ser15. The enhanced Ser15 can lead to the phosphorylation of Thr18. By suppressing the interaction of p53/mdm2 complex, Thr18 may protect p53 from ubiquitin-mediated degradation, which can stabilize and increase the protein expression of p53. When p53 was activated by AA, it could selectively activate target genes such as p21. The degree of up-regulation of p21 showed noticeable correlation with p53, which may because AA up-regulate p21 through activation of p53. The up-regulation of p21 protein can block the re-replication of DNA, sustain G2 arrest, and induce a G1/S arrest. Furthermore, p53 can directly decrease the likelihood of apoptosis of LNCaP cells by preventing their entry into the S phase.
The interaction between p53 and p300 is complex and controversial. The activation of p53-mediated target gene needs the auxiliary function of p300 and the related histone acetylation, which can enhance the stability of the p53-p300-DNA complexes and the affinity between the regulatory elements that affect gene transcription (30). p53 was also found in a stable complex with p300 by coimmunoprecipitation and gel supershift assay. p300 may act as coactivators of p53. But p53 can also be degraded by the ternary complex (p53/Mdm2/p300). In our experiments, after AA acted on LNCaP cells, the expression of p300 was significantly inhibited compared, whereas the expression of p53 increased. Therefore, AA can disrupt p300/p53 interaction and upregulate the p53.
In summary, AA inhibits p300 and AR, activates p53 and p21, and regulates other related target gene transcription. p300, AR, p53, p21 are all vital anti-tumor target gene for the treatment of prostate cancer. Therefore, AA has multiple anti-tumor activities and warrants further investigations.
Acknowledgements
Disclosure: The authors declare no conflict of interest.
References
- Gururajan M, Posadas EM, Chung LW. Future perspectives of prostate cancer therapy. Transl Androl Urol 2012;1:19-32.
- Britto AC, de Oliveira AC, Henriques RM, et al. In vitro and in vivo antitumor effects of the essential oil from the leaves of Guatteria friesiana. Planta Med 2012;78:409-14.
- de Melo JG, Santos AG, de Amorim EL, et al. Medicinal plants used as antitumor agents in Brazil: an ethnobotanical approach. Evid Based Complement Alternat Med 2011;2011:365359.
- Sowmyalakshmi S, Nur-E-Alam M, Akbarsha MA, et al. Investigation on Semecarpus Lehyam--a Siddha medicine for breast cancer. Planta 2005;220:910-8.
- Rea AI, Schmidt JM, Setzer WN, et al. Cytotoxic activity of Ozoroa insignis from Zimbabwe. Fitoterapia 2003;74:732-5.
- Devipriya B, Kumaradhas P. Probing the effect of intermolecular interaction and understanding the electrostatic moments of anacardic acid in the active site of p300 enzyme via DFT and charge density analysis. J Mol Graph Model 2012;34:57-66.
- Sukumari-Ramesh S, Singh N, Jensen MA, et al. Anacardic acid induces caspase-independent apoptosis and radiosensitizes pituitary adenoma cells. J Neurosurg 2011;114:1681-90.
- Hemshekhar M, Sebastin Santhosh M, Kemparaju K, et al. Emerging Roles of Anacardic Acid and Its Derivatives: A Pharmacological Overview. Basic Clin Pharmacol Toxicol 2011. [Epub ahead of print].
- Cui L, Miao J, Furuya T, et al. Histone acetyltransferase inhibitor anacardic acid causes changes in global gene expression during in vitro Plasmodium falciparum development. Eukaryot Cell 2008;7:1200-10.
- Ghizzoni M, Boltjes A, Graaf C, et al. Improved inhibition of the histone acetyltransferase PCAF by an anacardic acid derivative. Bioorg Med Chem 2010;18:5826-34.
- Sun Y, Jiang X, Chen S, et al. Inhibition of histone acetyltransferase activity by anacardic acid sensitizes tumor cells to ionizing radiation. FEBS Lett 2006;580:4353-6.
- Souto JA, Benedetti R, Otto K, et al. New anacardic acid-inspired benzamides: histone lysine acetyltransferase activators. Chem Med Chem 2010;5:1530-40.
- Souto JA, Conte M, Alvarez R, et al. Synthesis of benzamides related to anacardic acid and their histone acetyltransferase (HAT) inhibitory activities. Chem Med Chem 2008;3:1435-42.
- Sung B, Pandey MK, Ahn KS, et al. Anacardic acid (6-nonadecyl salicylic acid), an inhibitor of histone acetyltransferase, suppresses expression of nuclear factor-kappaB-regulated gene products involved in cell survival, proliferation, invasion, and inflammation through inhibition of the inhibitory subunit of nuclear factor-kappaBalpha kinase, leading to potentiation of apoptosis. Blood 2008;111:4880-91.
- Schultz DJ, Wickramasinghe NS, Ivanova MM, et al. Anacardic acid inhibits estrogen receptor alpha-DNA binding and reduces target gene transcription and breast cancer cell proliferation. Mol Cancer Ther 2010;9:594-605.
- Lee C, Zhang Q, Zi X, et al. TGF-β mediated DNA methylation in prostate cancer. Transl Androl Urol 2012;1:78-88.
- Ameri A, Alidoosti A, Hosseini Y, et al. Prognostic value of promoter hypermethylation of Retinoic Acid Receptor Beta (RARB) and CDKN2 (p16/MTS1) in prostate cancer. Chin J Cancer Res 2011;23:306-11.
- Ilic D, Forbes KM, Hassed C. Lycopene for the prevention of prostate cancer. Cochrane Database Syst Rev 2011;11:CD008007.
- Namiki M, Ueno S, Kitagawa Y. Role of hormonal therapy for prostate cancer: perspective from Japanese experiences. Transl Androl Urol 2012;1:160-72.
- Wolff JM, Schmid HP. Extension of the therapeutic spectrum in castration-resistant prostate cancer: Osteoclast inhibition with denosumab. Transl Androl Urol 2012;1:118-9.
- Palozza P, Colangelo M, Simone R, et al. Lycopene induces cell growth inhibition by altering mevalonate pathway and Ras signaling in cancer cell lines. Carcinogenesis 2010;31:1813-21.
- Limpens J, Schröder FH, de Ridder CM, et al. Combined lycopene and vitamin E treatment suppresses the growth of PC-346C human prostate cancer cells in nude mice. J Nutr 2006;136:1287-93.
- Chun JY, Tummala R, Nadiminty N, et al. Andrographolide, an herbal medicine, inhibits interleukin-6 expression and suppresses prostate cancer cell growth. Genes Cancer 2010;1:868-76.
- Sung B, Pandey MK, Ahn KS, et al. Anacardic acid (6-nonadecyl salicylic acid), an inhibitor of histone acetyltransferase, suppresses expression of nuclear factor-kappaB-regulated gene products involved in cell survival, proliferation, invasion, and inflammation through inhibition of the inhibitory subunit of nuclear factor-kappaBalpha kinase, leading to potentiation of apoptosis. Blood 2008;111:4880-91.
- Karamouzis MV, Konstantinopoulos PA, Papavassiliou AG. Roles of CREB-binding protein (CBP)/p300 in respiratory epithelium tumorigenesis. Cell Res 2007;17:324-32.
- Demarest SJ, Martinez-Yamout M, Chung J, et al. Mutual synergistic folding in recruitment of CBP/p300 by p160 nuclear receptor coactivators. Nature 2002;415:549-53.
- Wang X, Pan L, Feng Y, et al. p300 plays a role in p16(INK4a) expression and cell cycle arrest. Oncogene 2008;27:1894-904.
- Luo J, Li M, Tang Y, et al. Acetylation of p53 augments its site-specific DNA binding both in vitro and in vivo. Proc Natl Acad Sci U S A 2004;101:2259-64.
- Pan X, Zhao J, Zhang WN, et al. Induction of SOX4 by DNA damage is critical for p53 stabilization and function. Proc Natl Acad Sci U S A 2009;106:3788-93.
- Livengood JA, Scoggin KE, Van Orden K, et al. p53 Transcriptional activity is mediated through the SRC1-interacting domain of CBP/p300. J Biol Chem 2002;277:9054-61.