Antitumor effects of electrochemical treatment
Background
Cancer is a serious disease that causes about 13% of all deaths. According to statistics from the Health World Organization and the International Union Against Cancer in 2020 the number of new cases and deaths will increase to 16 and 10 million, respectively.
At present, different alternative therapeutic procedures are developing for this disease. Electrochemical treatment (EChT), also called electrotherapy (ET), is one of the alternative modalities for tumors treatment. It works when a low intensity direct electric current (DC) is applied to a tumor tissue through two or more electrodes placed within the tumor zone or in the surrounding areas. This treatment is noted for its great effectiveness, minimal invasiveness, local effect and low cost.
Actually, research on the DC antitumoral effects has increased enormously, which explains the growing number of studies carried out in this field (1-4). In a previous review, Nilsson et al. (5) give an overview of the experience related to EChT from year 1776 up to year 2000. However, our review summarizes the most important results and advances obtained in vitro and in vivo using EChT from year 2001 up to now. Also, we analyzed the present knowledge of the EChT destruction mechanism, and suggest some mechanisms and strategies from the thermodynamic point of view for this therapy.
Application of EChT in vitro models
We consider that in vitro experimental studies are very important to evaluate the direct effect of EChT on culture cell lines. In these studies, the electrodes are immersed into the culture medium (cells suspension or cell monolayer) and separated to the desired distance. This type of research allows us to describe the effects of an experimental variable. Also, it is appropriate for deducing a mechanism of action. Generally, this type of study allows more discernible results with less variables and amplified reactions.
Using this method, Yen et al. (1) evaluated the effectiveness of EChT in KB mammalian tumoral cells and investigated the factors that influenced the effectiveness of EChT. The results showed that there was a slowdown in cell growth when an amount of 0.3 C/mL (1.5 C for 5 mL of culture medium; 3V, 400 µA for 62.5 minutes) was applied. Also, an increase in EChT dose decreased the survival of tumoral cells. The researchers concluded that the toxic effect was related to the electric charge, the electric current intensity, and the length of the treatment. They also agreed that the pH effect on the cells is an important mechanism in EChT.
The cellular reactions generated by EChT treatment were evaluated by Veiga et al. (6,7) For these study the authors developed an experimental approach to assess the effects of the cathodic and anodic reactions separately. Additionally, Holandino et al. (8) used the same approach to analyze the electrolytic reactions. In this model, the cellular suspensions were distributed in a system of three acrylic chambers (2 mL of cell suspension per chamber), connected in series by filter-paper bridges and fitted with platinum electrodes at their extremities (Figure 1). In these conditions, an electric field of approximately 1V/cm was generated, and the cell suspensions could be exposed directly to the anodic reactions (AR), cathodic reactions (CR), and to an electric current without contact with the electrodes located in the intermediary chamber. The results obtained from this approach with various tumor cell types (mouse mastocytoma P815 cells, human promyelocytic leukemia HL-60 and human leukemic K562 cell line and its multidrug-resistant derivative K562-Lucena 1) show that the CR always caused necrotic cell lysis. However, the AR led to necrosis and apoptosis in its typical patterns (7). These results show that the products from the CR or AR influence the mechanism of cell death since the products induce damage to vital structures such as the mitochondria and the plasma membrane (6-8).
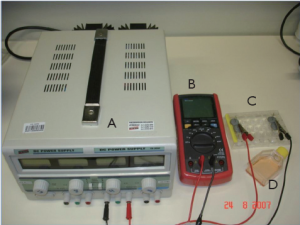
Recently, Bucco et al. (9), using the same experimental approach, study the effect of DC combinated with L-tyrosine nanoparticles on melanoma B16F10 cells. Cell suspensions were treated with of 2 mA, for 0 (control), 2, 4, 6, 8, and 10 min. The distance between the electrodes was 8 cm. In this study, in vitro cytotoxicity of DC was significantly increased when associated with L-tyrosine-loaded nanoparticles. This study showed that the combination of nanotechnology and DC enhanced the antitumoral effectiveness and offers a new perspective in cancer therapy.
Tumor regression of human leukemic cell lines (HL-60, ML-1, U-937, MOLT-4, EOL-1) was detected by Kurokawa et al. (10) using DC treatment during periods between 0-90 min and different intensities of EChT (0.03, 0.2, 0.5, 2, 4 and 5 mA). These authors detected apoptotic cell death characterized by cell shrinkage as well as nuclear and internucleosomal DNA fragmentation.
Tang et al. (11) evaluated the therapeutic effectiveness of EChT on Hela cells. The results showed that in the proximal area to the cathode there was a basic environment, and the levels of the K+, Ca2+ and Mg2+ cations had increased in this area. Concomitantly, the environment around the anode was acidic, and the Cl- anion was reduced. Furthermore, an increase in the percentage of cells in the G1 phase and a decrease of cells in the S phase of the cell cycle were observed. From this study the authors concluded that the antitumor effects of EChT were influenced by the degree of ionization, the pH level and cell cycle control.
In addition, Wartenberg et al. (12) analyzed the presence of apoptosis in tumor cells from the oral mucosa (UM-SCC-14-C) stimulated with DC fields (2 and 16 V/m for 24 hours). This research revealed a decrease in cell spread and an upregulation of the cyclin-dependent kinase (CDK). When an electric field of 4 V/m was applied for 24 hours, the level of apoptosis increased. The authors also reported a generation of reactive oxygen species (ROS) and the increased expression of the NADPH oxidase subunits as well as heat shock protein 70, which suggested that the apoptosis in tumor cells of the oral mucus was initiated by ROS generation in response to the DC treatment.
In vitro studies demonstrate that apoptosis is initiated by the generation of reactive oxygen species induced by DC. Also, these studies corroborate that alterations in cell ultrastructures induced by DC vary depending on the duration of therapy and electrodes polarity (6).
Application of EChT in animals models
A pioneer study by Chou et al. (13), using C3H/HeJ mice and Fisher 344 rats demonstrated that the insertion of electrodes at the tumor base significantly increased its destruction rate and decreased the damage to the body after the EChT was applied. Their report claimed that this method of inserting the electrodes and alternating the sequence of cathodes and anodes could cause a significant destruction at the tumor base. The researchers also determined the ratio of the number of electrodes to the size of the tumor, taking into account the effective area with necrosis around the electrodes (2 cm).
Ren et al. (14) investigated the influence of the space between the electrodes and dose by applying EChT to MCF-7 breast tumors in rats. The anatomical pathology study revealed that the therapy induced tumor necrosis. The authors concluded that the necrosis percentage increased significantly with the increasing dose. Also, this study showed that changes in electrode spacing (3, 5 and 10 mm) did not significantly affect the tumor responses to EChT within the same dose. However, Camué et al. (15) reported that this distance should be 0.7 cm, in agreement with the most used in DC treatment (1 cm). They also revealed an abrupt decrease of electric field intensity induced into the tumor for distances between electrodes below 0.28 cm, in agreement with the results of Ren et al. (14), who experimentally revealed the occurrence of short-circuiting and open-circuiting during DC treatment for 0.3 cm of distance between electrodes.
By other hand, in a very interesting study, Li et al. (16), using dog liver, proved the existence of a group of biochemical alterations around the anode and cathode in treated tumors. Around the anode researchers found a pH of 2, acid hemoglobin, tissue hydration, hydrogen ions that were the result of water electrolysis, and oxygen and chlorine gas emissions. From these emissions they explained the formation of hydrochloric acid and the acidic pH. At the cathode region the researchers found a pH of 12, tissue dehydration, and hydroxyl ions, which were the results of water electrolysis. Hydrogen gas emissions were also detected at the cathode, which provided the source of sodium hydroxide responsible for the basic pH. Halfway between the electrodes and in regions distal to the electrodes, no significant differences were found between the pH and the water concentration in tumors treated with EChT and those in the untreated tumors. Therefore, the authors concluded that the electrochemical effects of EChT occur around the electrodes, in agreement with the results reported by Tang et al. (11).
However, Von Euler et al. (17), analyzed the toxicity of pH changes as a mechanism for tumor cell death. Also, Von Euler et al. (18) studied similar macroscopic, histopathological and ultrastructural aspects in the liver by applying a four-electrode configuration (in Beagle dogs) and a two-electrode configuration (in Beagle dogs and in Sprague-Dawley rats). The doses applied were of 5, 10 and 90 C. In this study it was concluded that EChT cell toxicity could be clearly identified by histopathological and ultrastructural analyses. Also, this research showed that the distance between the electrodes and the polarity of these, are parameters that influence on the cellular damage.
In further studies carried out by Wemyss-Holden et al. (2) the effect of the local application of DC in the treatment of metastasis and tumors of the liver in pigs, was investigated. These researchers conducted the treatment changing the doses and the space between the electrodes to produce lesions of different sizes. The dose was established according to the volume of liver necrosis and a significant correlation was obtained between both parameters. The necrosis volume was smaller when the distance between the electrodes was reduced. Moreover, the liver enzymes only suffered temporary changes.
Ciria et al. (19) demonstrated that the EChT antitumor effects were increased when the electric charge dose increased and also a complete remission of the tumor was achieved. In this study Fibrosarcoma Sa-37 and Ehrlich tumors disappeared 60 days after treatment with an electric charge of 80 and 92 C/cm3 respectively, rendering Balb/c mice as cured. In this work they also found that the fibrosarcoma Sa-37 was more sensitive to EChT than the Ehrlich tumor. This finding confirmed the differences in the electrosensitivity between these types of tumors and proved that the more electrosensitive the tumor was, the more aggressive it was, which was previously suggested by Bergues et al. (20). These results indicate the existence of a specific threshold of DC for which each one of these tumors are completely destroyed. This hypothesis was theoretically corroborated by Bergues et al. (21).
In addition, previous studies conducted by Bergues et al. (22-27) showed that the type and size of a tumor have influence on the therapeutic effectiveness of EChT. They also demonstrated that the number of electrodes needed to apply EChT depended on the tumor size. Additionally, these researchs showed that the extension of tumor destruction is proportional to the number of electrodes placed. In these studies, EChT-treated tumors exhibited cell oedema, cell and organelle lysis, denaturation and coagulation of cytoplasmic proteins, and the presence of an important inflammatory reaction, indicative signs of a necrotic process.
Schroeppel et al. (28) evaluated the impact of EChT on fibrosarcoma tumor induced in Fischer 344 rats. This team of research applied 20 mA, over 66 minutes; and 10 mA during 16.6 minutes. Electrodes were inserted through the tumor base in a single plane, 4 mm apart, alternating anode and cathode. Tumors disappeared from all 16 treated rats within eight days. This group concluded that DC ablation provides highly effective tumor destruction. Also, they suggest that slower growth of tumors may involve the immune system.
Telló et al. (29-32) treated more than one hundred pet dogs and cats that had different types of cancers. The aim of these studies was to standardize the experiments in animals, to establish an adequate dose-response relationship and to determine the type of response of malignant tumors under EChT.
Telló’s research group evaluated the response of 27 dogs with mammary tumors of different volumes exposed to different DC charge intensities. This study showed the dose-response relationship presented by the dogs. The applied dose was ranged from 1-70 C/cm3. The animals’ complete response (absence of cancer cells) occurred at a DC dose between 21 and 70 C/cm3.
Also, Telló et al. (32) evaluated the EChT effect on animals with different types of tumors (such as: mammary, skin and subcutaneous tissue, head and neck). A continuous electric current of at least 20 C/cm3 was applied for 60 to 90 minutes through two or more electrodes (90% Platinum + 10% Rhodium) inserted 1-3 cm apart from one another in the cancerous region. Cytological exams of the tumors after EChT revealed development of inflammation and necrosis, with abundant macrophage infiltration as an early response. Infiltration of macrophages and lymphocytes increased after treatment, possibly due to antigen released from damaged tumor cells following EChT.
In these studies, Telló et al. (32) developed a new scheme of electrode placement called monopolar cerclage (Figure 2) with the aim of increase the effectiveness of the therapy. In this new scheme tumor volume is encircling with a metallic wire (cerclage wire) - analogous to a coil - and one pole of the DC current source is connected to a metallic/cerclage wire (which covers the tumor) while the other DC current source pole is connected outside of the tumor region. In this new electrode configuration, the negative (or positive) electrodes formed by cerclage wires are passed around the tumor volume and connected to a DC current source, while the positive (or negative) electrodes are connected to a metallic plate outside of the tumor region. According to the Brazilian research group, the EChT monopolar cerclage electrode configuration established an EChT standard protocol on the electrode distribution in the treated region.
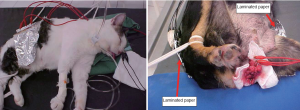
Thus, such results with animal models suggest that EChT is a method with local effect, clinically effective and safe. Also, studies in animal models corroborate that electrodes polarity, distance between electrodes, distribution of electrodes and the reactions that occur around each electrode are important parameters that have influence in therapy effectiveness.
Clinical application of EChT
In 1978 the clinical use of EChT in the treatment of malignant tumors in humans was reported for the first time when Nordenström (33) treated patients with lung cancer. In this study was explained the antitumor effects of EChT by means of mechanisms associated, such as pH alterations, electroosmotic transport of water, and the effects on ionic transmembrane flow. Since Nordenström developed his work in Sweden, the use of EChT has expanded to countries such as China, Germany, Japan, Korea, Australia, Slovenia, USA, Brazil, Cuba, Russia, and others, where this method has been used as an alternative for the treatment of patients with different histological types of tumors (5,34-44).
Since 1987 EChT has been used in China by Xin et al. (4) for the treatment of malignant and benign tumors, and up to date, it has been applied to over 20,000 patients. At present, this is the most complete clinical study that has been carried out. This study showed that EChT can be used in patients with inoperable malignant tumors or with tumors that do not respond to radio or chemotherapy, and also in patients with benign tumors, with satisfactory results in all cases. Also, Xin et al. (4) found that the effectiveness in the treatment of benign tumors was actually greater than in malignant tumors. This same result was obtained for superficial tumors as compared to visceral ones.
Liu et al. (45) researched the mechanism and effectiveness of EChT in 33 advanced stage cancer patients. Results showed that tumor cells in phases G1, G2/M, S, as well as the aneuploid cells, were destroyed. The researchers also found that the apoptotic proportion was very high after the therapy was applied. In this study it was concluded that this therapy could destroy tumor cells in a non-selective way by inducing apoptosis.
In 2005, Dr. Li Jing-Hong from the China-Japan Friendship Hospital supervised the use of EChT in Cuba for the treatment of patients with malignant and benign tumors. The study was developed by Verdecia et al. (46) to evaluate the effectiveness and safety of this method. Four patients with malignant tumors (ductal invasive carcinoma of the right breast; ductal invasive carcinoma of the left breast; liposarcoma of the posterior thoracic wall in the right scapular zone of the back and metastasis of an undifferentiated nasopharyngeal carcinoma) and one patient with benign tumor (congenital lymphangioma of the left ear) were treated. The applied dose was ranged from 40-100 mA. A significant reduction of tumor volume was obtained in all cases.
The therapy procedure applied in these patients showed that EChT is a safe method. A close follow-up of the treated patients demonstrated that the side effects were minimal. After the treatment was applied, the patients obtained a favorable rating in their physical and mental capacities and were in good general condition.
Clinical studies confirm the results obtained in vitro and in animal models. All these studies corroborate the effectiveness of EChT as well as the possibility of its use in tumors with different stages and locations (Table 1).
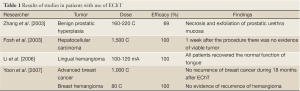
Full Table
The mechanisms involved in tumor death induced by EChT
Tumor cells are more susceptible to changes in their microenvironment than are normal cells, particularly those changes affecting the ionic composition of fluid surrounding the cells tissue. Precisely, the exposure of a tumor to DC can lead to a great variety of biochemical and physiological responses (47).
For this reason, several mechanisms have been proposed, such as: induction of bioelectrical forces into the tumor (26); Changes in tumor pH around electrodes (4,48); apoptosis induction (10,49); changes in tumor cells membrane potential (27,47); tumoral tissue ionization (48); production of toxic substances coming from electrochemical reactions (17); deposition of electrode material in tumor due to EChT aplication (48,50); immune system stimulation (27,28); extraction of water from tissue by electroosmosis (48,51); and simultaneous action of the electrochemical reactions that occur into the tumor (mainly involving reactive oxygen species) and strengthening the immune system (23).
This diversity of proposed mechanisms evidence that despite promising results obtained with EChT, there is no consensus among authors respect to the antitumor action mechanism. However, it is generally accepted that EChT induces electrolysis, electrophoresis, electroosmosis and electroporation in tumoral tissues, which cause chemical changes in the tumor cell microenvironment, and tumor metabolism. Also, is accepted that this alternative method promotes locally destructive electrolysis due to pH gradient (49,52-54).
These electrolytic reactions include generation of chlorine, oxygen and hydrogen ions at the anode and hydrogen gas and sodium hydroxide at the cathode (55).
As the electric current flows into electrodes, both tumor destruction and electrochemical reactions occurs basically at the surface of electrodes. An additional killing effect occurs as a result of water electrolysis and electrolytes yielding NaOH and HCl disseminate a certain distance from the electrode. Chloride ions accumulate around the anode will combine with H+ to form HCl. Na+ formed after electrolysis will move toward the cathode and combine with OH ions to form NaOH. However, chlorine causes some bleaching of the local tissue, whereas hydrogen produces local cavitation (48-50,56-61).
The electrolysis products make the region surrounding the anode very acidic (around pH 2) and the region surrounding the cathode strongly alkaline (pH 12). At these extreme pH values, the tissue proteins become denaturated and the cell structure collapses and the cell eventually dies. The pH changes in combination with the released chlorine gas and hydroxyl free radicals results in a locally cytotoxic microenvironment that induces coagulative and colliquative necrosis in the area of electrode application (48-50,56-62).
This necrosis could be explained from ischemia that appear in all DC treated tumors, which can lead to an irreversible damage of tumor cells and hence to cell death. Also, this necrosis could be associated with several experimental findings such as phospholipid degradation, loss of high-energy phosphate and increased intracellular calcium (6-8,17), membrane damage (13), ionic imbalance (48), mitochondrial alterations (56) and injury of the mechanism of ischemia/reperfusion (53,63). Precisely, the coagulative necrosis could be usually ischemic due to the presence of reactive oxygen species induced in the tumor by the electrochemical reactions, as demonstrated experimentally by Watenberg et al. (12).
An interesting fact is that tumor hyperacidification might activate cytolytic mechanisms through increased activity of lysosomes, resulting in the destruction of tumor tissues. Additionally, low pH values also inhibit glycolysis and protein synthesis upon which malignant tissues are dependent (64).
Furthermore, due to the existence electric field, an interstitial water flow from the anode to the cathode is generated, causing electroosmotic dewatering of the tumor tissue, which produce dehydration of the anodic site and hydration of the cathodic surface. This leads to an increase in tumor tissue turgor around the cathode which results in a resistance in blood flow and hence local ischaemia. During electroosmotic process all charged substances, dissolved or suspended in tissue, migrate in the electric field and as consequence an accumulation of ions and charged tissue constituents are obtained in different locations of the electric field (48-50,56-61).
The electric field influences the ion exchange across the cell membranes and thereby the conditions for many essential enzyme-regulated reactions. Also, EChT can produce a redistribution of cell surface receptors and influence the flow of specific ions through plasma membrane ion channels. By these reasons, EChT effectiveness in tumor cells could be influenced by the plasma membrane receptors and the electrical response level of those receptors; altered level in ion channel and Na+/H+ antiporter structure; and media pH variations. Any changes in the flow of ions through cellular ion channels can have significant effects on cellular metabolism, proliferation rate, cytoplasmic pH, mobility, cell cycle transitions, apoptosis, and inhibition of DNA synthesis (65-67).
By other hand, the possibility of DC in inducing an immune response or enhancing the toxicity of immune cells could be possible because of platelets and leukocytes are deposited around anode, due to its negative charge, forming micro-trombes in capillaries and blood vessels, near of the treatment zone. This fact produce an extensive embolism in blood vessels around the anode zone and oedema around cathode zone, which produce a blockage of microcirculation and tumor blood supply is interrupted due to an intense vasoconstriction. Also, oxyreductases in capillary endothelial cells could be activated by the superimposed electrical field and the free radicals produced in the lumen of capillaries could cause lysis of red blood cells with multiple capillary microthromboses occurring. These facts also could produce a decreased blood flow and also could contribute to the necrosis of tumor tissue (48-50,56-61).
If the applied electric field is sufficiently strong, the exposure to treatment can cause a significant increase in the electric conductivity and permeability of the cell plasma membrane and therefore all these severe disturbances produced by EChT in tumor cells cause its destruction rapidly and completely (47,50,55-57). Tumor, from the thermodynamic point of view, is a self-organizing dissipative structure which depends of the continuous flow of energy and matter from the host. By these reasons, in tumor cells the entropy production rate is always higher than that of healthy cells (68-71).
Tumor tissue exposure to an appropriate electric field may induce chemical and thermal changes due to electrochemical reactions and pH variations produced by EChT. All these changes alter the processes of structural and funtional self-organization in tumors causing its death (68-73). By other hand, this electric field may increase the entropy production in normal tissues above that of the tumor tissue. In this sense, the electric field can reverse the entropy flow leading to death of tumor cells, which is also enhanced by pH modifications induced by EChT (72).
However, when tumor is not completely destroyed by the action of the DC, a partial response to treatment is obtained. We consider that in this type of response to treatment, there are two stages (74). In the first stage, the tumor volume decreases up to a minimum value. In the second stage, tumor volume begins to rise again. The mechanism in the first stage can be explained because into the tumor are induced necrosis, apoptosis, chronic inflammation and vascular congestion due to DC action (19). In this case, in tumor zone appear polymorphonuclear leukocytes, monocytes, and toxic products coming from the electrochemical reactions (75,76). On the contrary, in the second stage the mechanism could be related to tumor self-organization. This fact could be explained because of tumor, as a complex- nonlinear dynamic system, is self-organized in time and space due to the dose of DC is not enough to induce the irreversible damage that leads to its complete destruction. This fact corroborates that tumor a structure far from thermodynamic equilibrium, and characterized by its high complexity, robustness and adaptability (77).
Self-organization is the capacity of a macromolecular complex to determine its own structure based on the functional interactions of its components. This process is an effective way to organize complex structures (69-78). Tumor could be considered as a self-organizing dissipative structure, characterized by the constant exchange of matter with the environment that surrounds it and its capability to a spontaneous transformation into a more complex system. Although this system presents a structural stability, however continuously generates entropy due to energy of dissipation. This structure remains stable by exporting this entropy into the environment (72,76).
Dosage and electrodes arrangement
All in vitro, in vivo and clinical studies discussed above show the diversity of points of views respect to the utilization mode and combination of the different parameters used in the EChT application. In these studies different doses of DC (intensity and time of application), number and position of electrodes, number of applications and variations of therapy are used (54,79). Indeed, the antitumor mechanisms of DC are proposed from the different experimental conditions established by each author. In each of these studies the use of different DC dose, exposure time and a specific configuration of electrodes are reported. This lack of consensus whith respect to the use of the therapy parameters and its lack of standardization has prevented its implementation in the Clinical Oncology as an alternative method.
It is important to note that for the selection of the optimal dose of DC and the arrangement of electrodes are required to consider many different variables, such as DC dose, exposure time of therapy, number of times to repeat the therapy, therapy variant (cathodic, anodic and anodic-cathodic therapy), mode of electrodes insertion (vertical or parallel to the larger diameter of tumor and radial), array of electrodes (number of electrodes, shape of the array, array size), tumor histological variety and type of host (74).
We consider that the lack of optimization of the array of electrodes in terms of its parameters can explain the diversity of electrode configurations and dose used in the different studies and thus the diversity of experimental results (Table 2). In our opinion, this parameter constitutes a major problem that has prevented the establishment of the EChT in Clinical Oncology.
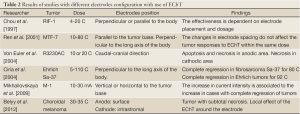
Full Table
The distribution of DC applied into the tumor depends on electrode array. This means that the antitumoral effectiveness of EChT depends on how the electrodes are placed into the tumor, with the aim to obtain the optimal distribution of applied DC, taking into account the dose (80).
The above mentioned justifies the importance of using mathematical models as an efficient and powerful tool to improve the distribution of electric field and electric current density into the tumor (80-85). This is an important fact for dose and treatment planning of EChT because of this tool allows to display the distributions of electric current density in the tumor and surrounding healthy tissue (two biological media with different conductivities). By other hand, mathematical models permit us the proper selection of the initial volume and the electrode configuration in order to increase the antitumor effectiveness the EChT. Additionally, this useful tool avoids unnecessary experiments and therefore excessive spending of time, material and human resources. However, in our opinion, mathematical models in the field of EChT in cancer have been exploited very little and much work remains to be done.
Conclusions
The results obtained in EChT application in vitro, in vivo, and clinic show that this is a simple, safe and effective therapy with minimal side effects because of its local character. Due to its favourable therapeutic profile, this therapy is a feasible treatment for cancer patients. Different electrode insertion methods and distribution have been used. Also, distinct currents, voltages and electric quantity have been empirically determined. Electrical dosage in tumors varies on depending of tumors conductivity, volume, position and electrode configuration. Also, the effectiveness of EChT depends on several factors such as: the electric charge dose (intensity and exposure time to EChT), the type of tumor (histological type, stage, initial size), electrode configuration (number, polarity, position), and EChT frequency (one or more stimuli). The great variety of antitumor mechanisms proposed for EChT shows that researchers have not reached a definitive conclusion on how EChT induces tumor tissue destruction. However, most scientists believe that tumor destruction is caused by the changes in pH and the action of the toxic products that result from the electrochemical reactions around the electrodes. These factors alone do not explain, however, neither the rapid destruction immediately after the EChT is applied, nor the extensive necrosis in time and space. Although the principle of EChT is simple, a standardized method is not yet available. The mechanism by which EChT affects tumor growth and survival may represent more complex process. For this reason, it is necessary to have a better understanding of the involved fundamental mechanisms and to elaborate a reliable strategy for the optimization of electrodes array and dose. At present, a useful tool employed in EChT standardization process is the mathematical modelling and analyze cancer as both disipative and complex system.
Acknowledgements
We thank Iliana Boza for their technical assistance and for Dr Marcio Lourenço Rodrigues for critical reading of the manuscript. We are grateful to Maria Cristina Lana Chaves de Castro for her technical assistance. This research was supported by the Ministry of Superior Education, Republic of Cuba, and by the Brazilian financial agencies (FAPERJ, CNPq and FUJB).
Disclosure: The authors declare no conflict of interest.
References
- Yen Y, Li JR, Zhou BS, et al. Electrochemical treatment of human KB cells in vitro. Bioelectromagnetics 1999;20:34-41.
- Wemyss-Holden SA, Dennison AR, Finch GJ, et al. Electrolytic ablation as an adjunct to liver resection: experimental studies of predictability and safety. Br J Surg 2002;89:579-85.
- von Euler H, Stråhle K, Thörne A, et al. Cell proliferation and apoptosis in rat mammary cancer after electrochemical treatment (EChT). Bioelectrochemistry 2004;62:57-65.
- Xin Y, Zhao H, Zhang W, et al. Electrochemical Therapy of Tumors. In: Rosch PJ, Markov MS. eds. Bioelectromagnetic Medicine. New York: Marcel Dekker Inc, 2004:709-26.
- Nilsson E, von Euler H, Berendson J, et al. Electrochemical treatment of tumours. Bioelectrochemistry 2000;51:1-11.
- Veiga VF, Holandino C, Rodrigues ML, et al. Cellular damage and altered carbohydrate expression in P815 tumor cells induced by direct electric current: an in vitro analysis. Bioelectromagnetics 2000;21:597-607.
- Veiga VF, Nimrichter L, Teixeira CA, et al. Exposure of human leukemic cells to direct electric current: generation of toxic compounds inducing cell death by different mechanisms. Cell Biochem Biophys 2005;42:61-74.
- Holandino C, Veiga VF, Rodrigues ML, et al. Direct current decreases cell viability but not P-glycoprotein expression and function in human multidrug resistant leukemic cells. Bioelectromagnetics 2001;22:470-8.
- de Campos VE, Teixeira CA, da Veiga VF, et al. L-tyrosine-loaded nanoparticles increase the antitumoral activity of direct electric current in a metastatic melanoma cell model. Int J Nanomedicine 2010;5:961-71.
- Kurokawa M, Sakagami H, Kokubu F, et al. Induction of apoptotic cell death by direct-current treatment in human leukemic cell lines. J Cancer Res Clin Oncol 1997;123:370-6.
- Tang B, Li L, Jiang Z, et al. Characterization of the mechanisms of electrochemotherapy in an in vitro model for human cervical cancer. Int J Oncol 2005;26:703-11.
- Wartenberg M, Wirtz N, Grob A, et al. Direct current electrical fields induce apoptosis in oral mucosa cancer cells by NADPH oxidase-derived reactive oxygen species. Bioelectromagnetics 2008;29:47-54.
- Chou CK, McDougall JA, Ahn C, et al. Electrochemical treatment of mouse and rat fibrosarcomas with direct current. Bioelectromagnetics 1997;18:14-24.
- Ren RL, Vora N, Yang F, et al. Variations of dose and electrode spacing for rat breast cancer electrochemical treatment. Bioelectromagnetics 2001;22:205-11.
- Camué H, Cabrales LE, Aguilera AR, et al. Influence of electrode array parameters used in electrotherapy on tumor growth kinetics: A mathematical simulation. Math Comput Simul 2012;82:1396-406.
- Li K, Xin Y, Gu Y, et al. Effects of direct current on dog liver: possible mechanisms for tumor electrochemical treatment. Bioelectromagnetics 1997;18:2-7.
- von Euler H, Söderstedt A, Thörne A, et al. Cellular toxicity induced by different pH levels on the R3230AC rat mammary tumour cell line. An in vitro model for investigation of the tumour destructive properties of electrochemical treatment of tumours. Bioelectrochemistry 2002;58:163-70.
- von Euler H, Olsson JM, Hultenby K, et al. Animal models for treatment of unresectable liver tumours: a histopathologic and ultra-structural study of cellular toxic changes after electrochemical treatment in rat and dog liver. Bioelectrochemistry 2003;59:89-98.
- Ciria HC, Quevedo MS, Cabrales LB, et al. Antitumor effectiveness of different amounts of electrical charge in Ehrlich and fibrosarcoma Sa-37 tumors. BMC Cancer 2004;4:87.
- Bergues Cabrales LL, Ciria LC, de la Guardia Peña O, et al. Efecto Antitumoral de la corriente eléctrica directa: estudio en tumores de Ehrlich y fibrosarcoma Sa-37. Rev Cub Oncol 2001;17:176-9.
- Cabrales LE, Aguilera AR, Placeres RJ, et al. Mathematical modeling of tumor growth in mice following low-level direct electric current. Math Comp Simul 2008;78:112-20.
- Cabrales LB, Aldana RH, Bruzón RP, et al. Efectos de la corriente eléctrica directa en el tumor murino subcutáneo de Ehrlich. I: Estudios de necrosis y volumen del tumor en estadíos avanzados. Rev Bras Cancerol 1998;44:203-10.
- Cabrales LB, Ciria HC, Bruzón RP, et al. Electrochemical treatment of mouse Ehrlich tumor with direct electric current. Bioelectromagnetics 2001;22:316-22.
- Camué HC, Cabrales LB, Suárez Quevedo O, et al. Efectos de la CED en el crecimiento del tumor de Ehrlich. Rev Cub Oncol 2001;17:167-70.
- Bergues LC, Gómez LL. La Electroterapia: una alternativa terapéutica para el tratamiento de tumores. Rev Cub Med 2003;42:6.
- Suárez CQ, Pérez PR, Bergues CL, et al. Alteraciones inducidas en el fibrosarcoma Sa-37 por la acción citotóxica de la corriente eléctrica directa. Rev Cub Med 2003;42:4.
- Bergues LC. La electroterapia una nueva alternativa para el tratamiento de tumores malignos. Estudios preclínicos. Tesis de Doctorado. Universidad de Oriente. Cuba 2003.
- Schroeppel EA, Kroll K, Damon MC, et al. Direct current ablation destroys multi-stage fibrosarcomas in rats. Conf Proc IEEE Eng Med Biol Soc 2009;2009:3099-104.
- Telló M, Dias G, Cardona A, et al. Tumor Compression Due Application of DC Current. IEEE T Magn 2001;37:3753-6.
- Telló M. Uso da Corrente Elétrica no Tratamento do Câncer. EDIPUCRS: Pontificia Universidade Católica do Rio Grande do Sul Press. 2004.
- Parise O, Oliveira LO, Telló M, et al. Electrolysis and Electrochemical Therapy in the Treatment of Spontaneous Head and Neck Tumors of Cats and Dogs. Annual Meeting & Research Workshop on the Biology, Prevention and Treatment of Head and Neck Cancer. 2006.
- Telló M, Oliveira L, Parise O, et al. Electrochemical therapy to treat cancer (in vivo treatment). Conf Proc IEEE Eng Med Biol Soc 2007;2007:3524-7.
- Nordenström B. Preliminary clinical trials of electrophoretic ionization in the treatment of malignant tumors. IRCS Med Sci 1978;6:537-40.
- Wang HL. Electrochemical therapy of 74 cases of liver cancer. Eur J Surg Suppl 1994;(574):55-7.
- Plesnicar A, Sersa G, Vodovnik L, et al. Electric treatment of human melanoma skin lesions with low level direct electric current: an assessment of clinical experience following a preliminary study in five patients. Eur J Surg Suppl 1994;(574):45-9.
- Xin YL. Organisation and spread of electrochemical therapy (ECT) in China. Eur J Surg Suppl 1994;(574):25-9.
- Lao YH, Ge TG, Zheng XL, et al. Electrochemical therapy for intermediate and advanced liver cancer: a report of 50 cases. Eur J Surg Suppl 1994;(574):51-3.
- Xin Y, Xue F, Ge B, et al. Electrochemical treatment of lung cancer. Bioelectromagnetics 1997;18:8-13.
- Wu G, Zhou X, Huang M. Electrochemical therapy and implanted ports treatment for unresectable carcinoma of body and tail of pancreas. Zhonghua Wai Ke Za Zhi 2001;39:596-8.
- Fosh BG, Finch JG, Lea M, et al. Use of electrolysis as an adjunct to liver resection. Br J Surg 2002;89:999-1002.
- Fosh BG, Finch JG, Anthony AA, et al. Use of electrolysis for the treatment of non-resectable hepatocellular carcinoma. ANZ J Surg 2003;73:1068-70.
- Zhang M, Gong K, Li N, et al. Transurethral electrochemical treatment of benign prostatic hyperplasia. Chin Med J (Engl) 2003;116:104-7.
- Li JH, Xin YL, Zhang W, et al. Effect of electro-acupuncture in treating patients with lingual hemangioma. Chin J Integr Med 2006;12:146-9.
- Yoon DS, Ra YM, Ko, DG, et al. Introduction of Electrochemical Therapy (EChT) and Application of EChT to The Breast Tumor. J Breast Canc 2007;10:162-8.
- Liu J, Wei Y, Luo F, et al. Cell cycle analysis and pathological changes of malignant tumors treated with electrochemical therapy. Hua Xi Yi Ke Da Xue Xue Bao 2000;31:104-6.
- Jarque MV, Mateus MA, Li JH, et al. Primeras experiencias clínicas en Cuba sobre el uso de la electroterapia en cuatro pacientes con tumores sólidos malignos superficiales. MEDISAN 2007;11:1.
- Kotnik T, Miklavcic D. Theoretical evaluation of voltage inducement on internal membranes of biological cells exposed to electric fields. Biophys J 2006;90:480-91.
- Vijh AK. Phenomenology and Mechanisms of Electrochemical Treatment (ECT) of Tumors. Mod Aspect Electric 2006;39:231-74.
- Gu Y, Kang KM, Hasegawa T, et al. Electrochemical cancer therapy induces apoptosis in SCC-7 tumor of mice. Journal of the IABC 2003;1:22-32.
- Kim HB, Ahn S, Jang HJ, et al. Evaluation of corrosion behaviors and surface profiles of platinum-coated electrodes by electrochemistry and complementary microscopy: biomedical implications for anticancer therapy. Micron 2007;38:747-53.
- McLaughlin S, Poo MM. The role of electro-osmosis in the electric-field-induced movement of charged macromolecules on the surfaces of cells. Biophys J 1981;34:85-93.
- Dodd NJ, Moore JV, Taylor TV, et al. Preliminary evaluation of low level direct current therapy using magnetic resonance imaging and spectroscopy. Phys Med 1993;9:285-9.
- Griffin DT, Dodd NJ, Moore JV, et al. The effects of low-level direct current therapy on a preclinical mammary carcinoma: tumour regression and systemic biochemical sequelae. Br J Cancer 1994;69:875-8.
- Mikhailovskaya AA, Kaplan MA, Brodskij RA, et al. Evaluation of antitumor efficiency of electrochemical lysis on the model of M-1 sarcoma. Bull Exp Biol Med 2009;147:91-3.
- Samuelsson L, Jönsson L. Electrolyte destruction of lung tissue. Electrochemical aspects. Acta Radiol Diagn (Stockh) 1980;21:711-4.
- Holandino C, Veiga VF, Capella MM, et al. Damage induction by direct electric current in tumoural target cells. Indian J Exp Biol 2000;38:554-8.
- Mycielska ME, Djamgoz MB. Cellular mechanisms of direct-current electric field effects: galvanotaxis and metastatic disease. J Cell Sci 2004;117:1631-9.
- Hinz S, Egberts JH, Pauser U, et al. Electrolytic ablation is as effective as radiofrequency ablation in the treatment of artificial liver metastases in a pig model. J Surg Oncol 2008;98:135-8.
- Schaefer N, Schafer H, Maintz D, et al. Efficacy of direct electrical current therapy and laser-induced interstitial thermotherapy in local treatment of hepatic colorectal metastases: an experimental model in the rat. J Surg Res 2008;146:230-40.
- Salzberg M, Kirson E, Palti Y, et al. A pilot study with very low-intensity, intermediate-frequency electric fields in patients with locally advanced and/or metastatic solid tumors. Onkologie 2008;31:362-5.
- Berry D, Garcea G, Chong C, et al. Systematic reaction to electrolytic treatment of pig livers in vivo. ANZ J Surg 2004;74:586-90.
- Czymek R, Dinter D, Löffler S, et al. Electrochemical treatment: An investigation of dose-response relationships using an isolated liver perfusion model. Saudi J Gastroenterol 2011;17:335-42.
- Jarm T, Wickramasinghe YA, Deakin M, et al. Blood perfusion of subcutaneous tumours in mice following the application of low-level direct electric current. Adv Exp Med Biol 1999;471:497-506.
- Oji C, Ani J. Destruction of an advanced malignant tumour by direct electrical current-case report. Health 2010;2:1049-53.
- Lyte M, Gannon JE, O’Clock GD Jr. Effects of in vitro electrical stimulation on enhancement and suppression of malignant lymphoma cell proliferation. J Natl Cancer Inst 1991;83:116-9.
- O’Clock GD. The Effects of In Vitro Electrical Stimulation on Eukaryotic Cells: Suppression of Malignant Cell Proliferation. J Ortho Med 1997; 12:173-81.
- O’Clock GD, Leonard T. In Vitro Response of Retinoblastoma, Lymphoma and Non-Malignant Cells to Direct Current: Therapeutic Implications. Deutsche Zeitschrift für Onkologie (German Journal of Oncology) 2001;33:85-90.
- Klimek R. Biology of cancer: thermodynamic answers to some questions. Neuro Endocrinol Lett 2001;22:413-6.
- Patiño JF. Oncología, caos, sistemas complejos adaptativos y estructuras disipativas. Rev Colomb Cir 2002;7:1-9.
- Waliszewski P. A principle of fractal-stochastic dualism and Gompertzian dynamics of growth and self-organization. Biosystems 2005;82:61-73.
- Luo LF. Entropy Production in a Cell and Reversal of Entropy Flow as an Anticancer Therapy. Front Phys China 2009;4:122-136.
- Molnar J, Varga ZG, Thornton-Benko E, et al. The Second Law of Thermodynamics and Host-Tumor Relationships: Concepts and Opportunities, Application of Thermodynamics to Biological and Materials Science, Prof. Mizutani Tadashi (Ed.), 2011:204-26.
- Davies PC, Demetrius Ll, Tuszynski JA. Cancer as a dynamical phase transition. Theor Biol Med Model 2011;8:30.
- Camué HC. Volumen tumoral y densidad de corriente eléctrica generada por un sistema de arreglos de electrodos con corriente eléctrica directa: estudio experimental y teórico. Tesis de Doctorado. Universidad de Oriente. Cuba (2010).
- Simcic S, Bobanović F, Kotnik V, et al. Local changes in membrane potential intensify neutrophil oxidative burst. Physiol Chem Phys Med NMR 1997;29:39-47.
- Bobanovič F, Klampfer L, Miklavčič D. The effect of direct current on NK cell activity. Trans BRAGS 1991;11-20.
- Izquierdo E, Alonso E, Nieto JM. Entropy Production Rate for Avascular Tumor Growth. Journal of Modern Physics 2011;2:615-20.
- Menchón S. Modelado de las diversas etapas del crecimiento del cáncer y de algunas terapias antitumorales. Tesis de Doctorado. Universidad Nacional de Córdoba. Argentina (2007).
- Belyy Y, Tereshchenko A, Shatskih A. Electrochemical lysis of an intraocular tumour using a combination of electrode placements. Ecancermedicalscience 2012;6:272.
- Turjanski P, Olaiz N, Abou-Adal P, et al. pH front tracking in the electrochemical treatment (EChT) of tumors: Experiments and simulations. Electrochimica Acta 2009;54:6199-206.
- Aguilera AR, Cabralesb LE, Ciriab HM, et al. Electric current density distribution in planar solid tumor and its surrounding healthy tissue generated by an electrode elliptic array used in electrotherapy. Math Comput Simul 2010;80:1886-902.
- Kotnik T, Miklavcic D. Theoretical evaluation of the distributed power dissipation in biological cells exposed to electric field. Bioelectromagnetics 2000;21:385-94.
- Corović S, Pavlin M, Miklavcic D. Analytical and numerical quantification and comparison of the local electric field in the tissue for different electrode configurations. Biomed Eng Online 2007;6:37.
- Olaiza N, Magliettia F, Suárez C, et al. Electrochemical treatment of tumors using a one-probe two-electrode device. Electrochimica Acta 2010;55:6010-4.
- Colombo L, González G, Marshall G, et al. Ion transport in tumors under electrochemical treatment: in vivo, in vitro and in silico modeling. Bioelectrochemistry 2007;71:223-32.